How Magnets Work? Magnets have fascinated humanity for centuries, drawing curiosity with their invisible yet powerful force. But what exactly makes magnets stick to certain metals? Why are neodymium magnets so much stronger than the ceramic magnets you might remember from science class? To understand these questions, we first need to define what a magnet is.
Table of Contents
What is a Magnet?
A magnet is an object that produces a magnetic field, attracting materials like iron, nickel, and cobalt. Magnetic fields are represented as lines of force that exit from the magnet’s north pole and enter its south pole. There are three primary types of magnets:
- Permanent Magnets (Hard Magnets): These magnets create their own magnetic field all the time. Examples include neodymium, samarium cobalt, and ceramic magnets. They are used in everyday items like speakers, hard drives, and even electric motors due to their ability to maintain a consistent magnetic field.
- Temporary Magnets (Soft Magnets): These only produce a magnetic field when in the presence of a stronger magnet or electric current. Iron nails that stick to a magnet temporarily are an example. They are commonly used in applications like electromagnets and magnetic locks where the magnetic effect needs to be switched on and off.
- Electromagnets: These generate magnetic fields only when electricity passes through them. Made by wrapping a wire around a metal core and passing a current through it, electromagnets are used in MRI machines, maglev trains, and industrial cranes to lift heavy metal objects.
How Magnets Work
The key to understanding how magnets work lies in the behavior of electrons inside the material. Electrons are tiny charged particles that spin around the nucleus of an atom. This spinning creates a small magnetic field. In most materials, the magnetic fields of electrons cancel out because they spin in opposite directions. However, in magnetic materials like iron, cobalt, and nickel, many of these electron spins align in the same direction, creating larger magnetic fields that work together to form a magnet.

When you bring a magnet close to a magnetic material, the magnetic domains within that material start to align with the magnetic field of the magnet. This alignment strengthens the overall magnetic field, making the material itself act like a magnet temporarily. This is how magnets work to attract objects.
The strength of a magnet depends on how well these domains are aligned. When the domains are randomly oriented, the material is not magnetic. When they are aligned, the magnetic force becomes strong and noticeable. This alignment can be influenced by applying an external magnetic field or even by physically striking the material while it’s in a magnetic field.
Quantum Mechanics and Magnetism
At the microscopic level, magnetism is rooted in quantum mechanics. Electrons in atoms behave as tiny magnets due to their charge and spin. In most materials, electrons spin in opposite directions, canceling out their magnetic properties. However, in elements like iron, cobalt, and nickel, unpaired electrons spin in the same direction, allowing the material to become strongly magnetic. This alignment of electron spins is what gives ferromagnetic materials their unique magnetic properties.

Types of Magnetic Materials
- Ferromagnetic materials: First, we have ferromagnetic materials, which are strongly attracted to magnets. Imagine a powerful magnet that pulls things like iron, nickel, or cobalt toward it. These materials can even become magnets themselves. Inside them, there are tiny regions called magnetic domains. Think of these domains like little “mini magnets” inside the material that align when exposed to a magnetic field, giving the material strong magnetic properties.
- Paramagnetic materials: Then, there are paramagnetic materials. These materials, like aluminum and platinum, are only weakly attracted to magnets. But here’s the catch: they only show this attraction when they are near a magnet or within a magnetic field. As soon as the field is removed, the attraction disappears, making their magnetic properties temporary and not as strong as ferromagnetic materials.
- Diamagnetic materials: Next, we have diamagnetic materials, such as copper and gold. These materials don’t get attracted to magnets at all. In fact, they slightly repel them! When exposed to a magnetic field, they create a tiny opposing magnetic field, making them push away from the magnet. This is why they’re considered “anti-magnetic” in a way.
- Antiferromagnetic materials: Finally, there are antiferromagnetic materials, which are a bit trickier. In these materials, like manganese oxide, the tiny magnetic moments of the atoms inside them align in opposite directions. It’s like each atom’s magnetic “north pole” is canceled out by its “south pole,” which makes the material not magnetic overall. This alignment prevents them from being attracted to a magnet.
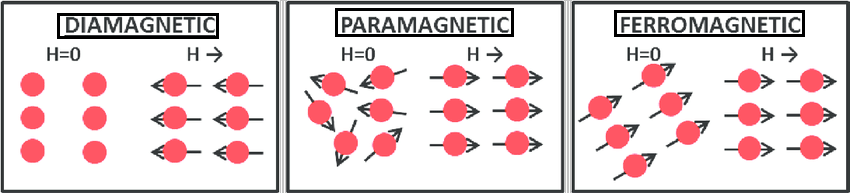
Magnetic Domains and Hysteresis
In ferromagnetic materials, tiny regions called magnetic domains exist where the magnetic moments are aligned. When a material is magnetized, these domains align in the same direction, creating a strong magnetic field. This alignment process can be visualized using a hysteresis loop, which shows the relationship between the magnetic field strength and magnetization. The ability of a material to retain magnetization even after the external magnetic field is removed is called remanence.
How Magnets are Made
Creating a magnet involves aligning the magnetic domains. This can be done by:
1. Placing the Material in a Strong External Magnetic Field
How It Works:
- When a ferromagnetic material (like iron, nickel, or cobalt) is exposed to a strong external magnetic field, the magnetic domains inside begin to reorient themselves.
- Initially, domains point in random directions, canceling each other out (no net magnetism).
- The external field exerts torque on the domains, forcing them to align parallel to the field.
- As the field strength increases, more and more domains flip until nearly all are aligned (saturation magnetization).
Key Factors:
- Field Strength (H): A stronger field aligns domains more effectively.
- Material Properties: Soft magnetic materials (like iron) align easily but lose magnetism quickly. Hard magnetic materials (like neodymium) resist alignment but retain magnetism longer.
- Temperature: Heating reduces alignment (domains randomize at the Curie temperature).
Applications:
- Manufacturing permanent magnets (e.g., Alnico, ferrite magnets).
- Degaussing (erasing magnetic fields by applying an alternating field that randomizes domains).
2. Physically Striking the Material While Aligned (Hammering or Vibration)
How It Works:
- Even after exposure to a magnetic field, some domains may resist alignment due to crystal defects or stress.
- Mechanical shock (hammering, vibration) provides energy to help domains overcome these barriers.
- Historically, blacksmiths would heat and hammer iron bars while aligning them north-south to make compass needles.
Key Factors:
- Direction Matters: Striking while the material is in a magnetic field ensures domains align with the field.
- Grain Structure: Crystalline materials (like steel) respond better than amorphous metals.
- Work Hardening: Excessive hammering can introduce defects that hinder magnetization.
Applications:
- Early compass manufacturing.
- Strengthening magnetic cores in transformers.
3. Passing an Electric Current Through It (Electromagnetization)
How It Works:
- When an electric current flows through a wire, it generates a circular magnetic field around it (Ampère’s Law).
- If the wire is coiled around a ferromagnetic core (like iron), this field penetrates the material and aligns its domains.
- The strength depends on:
- Current (I): More current = stronger field.
- Number of Coils (N): More loops = stronger magnetization.
- Core Material: Soft iron magnetizes quickly; steel retains magnetism longer.
Key Factors:
- AC vs. DC:
- DC current creates a steady field (good for permanent magnetization).
- AC current can demagnetize if slowly reduced (used in degaussing).
- Core Permeability: High-permeability materials (like mu-metal) enhance magnetization.
Applications:
- Electromagnets (used in MRI machines, speakers, relays).
- Magnetizing tools (e.g., screwdrivers, pickups in electric guitars).
Comparison of Magnetization Methods
Method | How It Works | Best For | Limitations |
---|---|---|---|
External Magnetic Field | Forces domain alignment via strong field | Permanent magnets, industrial magnetization | Requires very strong fields for hard materials |
Hammering/Vibration | Mechanical energy helps domains overcome resistance | Historical methods, fine-tuning magnetization | Can introduce defects if overdone |
Electric Current | Current-induced field aligns domains | Electromagnets, controllable magnetization | Requires power source; heat buildup possible |
Why Magnets Stick to Some Materials
Magnets are attracted to materials that have unpaired electrons that spin in the same direction. In metals like iron, the orbital magnetic moment encourages nearby atoms to align along the same north-south field lines. Iron and other ferromagnetic materials are crystalline. As they cool from a molten state, groups of atoms with parallel orbital spin line up within the crystal structure, forming magnetic domains.
Properties of Magnets Explained
1. Two Poles That Can’t Be Separated
- Every magnet must have both north and south poles (like two sides of a coin)
- If you break a magnet in half, each piece becomes a new complete magnet with its own N and S poles
- No magnetic monopoles exist in nature (unlike electric charges where + and – can separate)
Example:
Break a bar magnet → get two smaller bar magnets, each with N+S poles
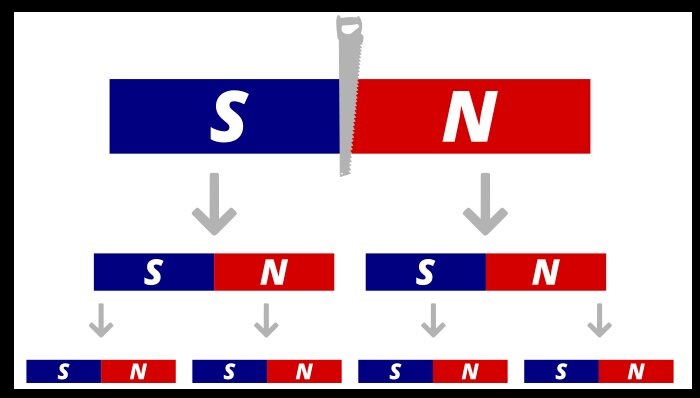
2. Opposite Poles Attract, Like Poles Repel
- N and S poles pull toward each other
- N-N or S-S poles push apart (try it with two fridge magnets!)
- This is why compass needles align with Earth’s magnetic field
Visualization:
Imagine two magnets as friends:
✓ N+S = Best friends who hug
✗ N+N or S+S = Enemies who push away
3. Magnetic Field Lines (Invisible Force)
- Magnets create an invisible force field around them
- Field lines:
- Exit from North pole
- Enter at South pole
- Never cross each other
- Strongest at the poles (where iron filings cluster)
Experiment:
Place paper over a magnet and sprinkle iron filings to see the pattern!

4. Magnetic navigation
The suspended magnet’s North Pole points toward the Earth’s Magnetic North, while its South Pole points toward the Magnetic South. This is the basic principle behind how compasses work. Ancient explorers used this concept to navigate across the seas long before modern technology was invented.
This natural alignment occurs because the Earth’s core generates magnetic forces that interact with the suspended magnet, causing it to seek equilibrium along the magnetic field lines.
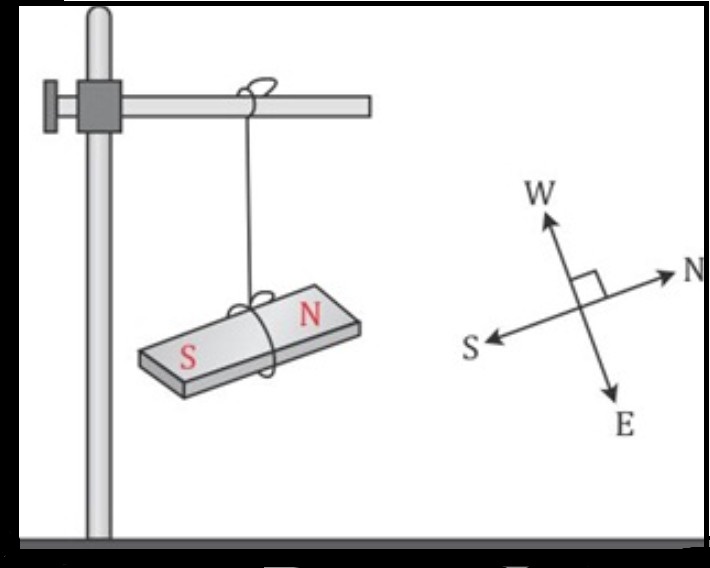
The Earth’s Magnetic Field
The Earth generates its own magnetic field, known as the geomagnetic field, primarily created by the movement of molten iron and nickel in its outer core. This field extends into space and protects us from solar winds and cosmic radiation.
Applications of Magnets
- Electric Motors and Generators
- Data Storage
- Speakers and Microphones
- MRI Machines
- Magnetic Levitation
- Compasses
Magnetic Levitation: The Future of Transport
One of the most fascinating applications of magnets is in magnetic levitation (maglev). This technology allows trains to float above tracks, eliminating friction and allowing for incredibly smooth and fast travel. Powerful electromagnets lift the train, while others propel it forward. Countries like Japan and China are already using maglev trains, reaching speeds of over 600 km/h.Applications of Magnets
1. Electric Motors and Generators:
Magnets play a key role in electric motors and generators. In these devices, magnets interact with electric currents to produce mechanical motion (in motors) or generate electric current (in generators). By rotating a coil of wire within a magnetic field, electricity is generated, which powers devices ranging from household appliances to large industrial machines.
2. Data Storage:
Magnets are used extensively in data storage technologies. Hard drives, for example, store data on magnetic disks. The magnetic field of a tiny read/write head moves across the disk’s surface, changing the magnetic alignment of bits to store or retrieve information. This is how millions of files can be saved on your computer’s hard drive.
3. Speakers and Microphones:
Magnets are essential components of speakers and microphones. In a speaker, the interaction between the magnetic field of a magnet and an electric current in a coil creates sound vibrations. In microphones, the movement of a diaphragm within a magnetic field generates an electrical signal, which can then be amplified into sound.
4. MRI Machines:
Magnetic Resonance Imaging (MRI) machines use powerful magnets to generate high-resolution images of the inside of the body. The magnetic fields align the hydrogen atoms in the body, and then a radiofrequency pulse is used to disturb this alignment. As the atoms return to their original positions, they release energy that is captured and used to form detailed images of organs and tissues.
5. Magnetic Levitation (Maglev):
Magnetic levitation is used in transportation systems like maglev trains. These trains float above the tracks using magnets, eliminating friction and allowing them to travel at extremely high speeds. This technology has the potential to revolutionize transportation by offering fast, smooth, and efficient travel options.
6. Compasses:
Compasses use magnets to determine direction. The needle of a compass is magnetized, and as the Earth itself is a giant magnet, the needle aligns itself with the Earth’s magnetic field. This allows travelers and navigators to determine the direction of the magnetic poles, aiding in navigation.
Fun Facts About Magnets
- Magnetic fields surround the Earth: The Earth itself acts like a giant magnet, and its magnetic field is responsible for the Northern and Southern Lights (Aurora Borealis).
- Magnetic forces can influence light: In certain extreme conditions, such as in the presence of a strong magnetic field, light can be bent, changing the way it behaves.
- Neodymium magnets are the strongest known magnets: These magnets, which are commonly used in modern technology like hard drives and motors, are made from an alloy of neodymium, iron, and boron.
- Magnets can lose their magnetism: If a magnet is heated too much or struck forcefully, it can lose its magnetism. This happens because the heat or impact can disrupt the alignment of the atoms’ magnetic domains.
Conclusion
Magnetism is a fascinating phenomenon that affects many aspects of our daily lives, from the devices we use to the technology that powers industries. Understanding how magnets work helps us appreciate the role they play in shaping the modern world. Whether it’s in the form of a permanent magnet, an electromagnet, or a temporary magnet, the magnetic force is crucial in various technologies, including data storage, healthcare, and transportation. By continuing to study magnetism, scientists and engineers can unlock even more advanced uses for this invisible yet powerful force.
Do you want to read more about science. check this out: 1] What is Gravity? 2] What is Electricity? 3] What Causes Earthquakes? 4] Why is the Sky Blue? 5] How Do Plants Grow?